It’s no secret that stem cell (SC) research has taken off over the past decade. Stem cells from bone marrow are being used to craft a cartilage patch for knee injury. A new company wants to take patient skin cells and turn them into dopamine neuron precursors in Parkinson’s patients. And a pig-derived decellularized hydrogel was shown to support the growth of SC organoids, which brings researchers a step closer to organoid transplants in a clinical setting (synthetic gels are considered unsuitable for use in humans).
Cells that are capable of becoming any other cell type in the body, and that when combined with cutting-edge 3D technology have the potential to form entire organs, embody the ultimate paradigm shift; doctors would be able to treat myriad devastating diseases. Organ transplant lists would become archaic and unnecessary. But we’re not there just yet. And in the meantime, scientists are troubleshooting ways to deal with common challenges still associated with SC work.
Getting stem cells to the clinic driving change
Historical culture methods relied on the support of animal-derived products, explains Mindy Goldsborough, chief scientific officer at ATCC. “Interest in using stem cells in therapeutic approaches has driven significant changes in culture methods.” Newer products and protocols use feeder-free and serum-free culture methods. This approach significantly reduces the variability associated with reagents, such as serum, that often see lot-to-lot differences.
Xinying Xie, North America technical liaison and applications scientist at Corning Life Sciences, explains that feeder cells can be replaced with extracellular matrices such as Matrigel® matrix, rLaminin-521 (human), or Synthemax™ II-SC, “along with the fine-tuning of medium formulations of essential amino acids, salts, other nutritional elements, and growth factors.” Further, chemically defined media that relies on only recombinant proteins and hormones can create a more controlled environment.
“With these types of advancements, the ability to scale production to necessary levels for therapeutic purposes became more of a reality and the regulatory path became less burdensome due to xeno-free culture conditions,” adds Goldsborough.
Sheela Jacob, scientist at ATCC, echoes Goldsborough. She says that culturing induced pluripotent stem cells (iPSCs) required coculture with fibroblast feeders or serum-free medium to prevent differentiation. But this complicates the production process. Not only is it difficult to remove xenobiotic cells, but “murine pathogens may contaminate the final, differentiated product.” As such, ATCC developed an iPSC production system that is free of these components. “This culture system is high performing: iPSCs cultured in this system exhibit healthy morphology, proliferate at an appropriate rate, and continue in their pluripotent state for over 30 passages,” notes Jacob.
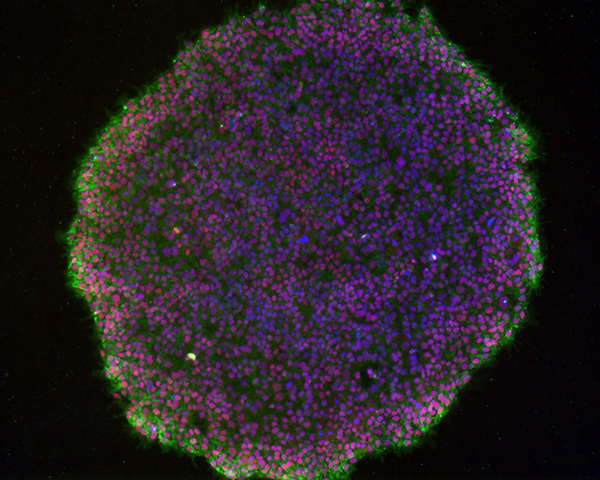
Image: Characteristic morphology of iPSCs grown in feeder-free cultures. Image courtesy of ATCC.
In the quest for ultra-pure reagents that help increase SC culture reproducibility and consistency, some companies are turning to barley. Anne Sloan, technical support scientist at Cell Sciences, says that the company offers 20 growth factors and cytokines using a barley expression system. “With its specialized endosperm storage tissue, [it] has unique features such as eukaryotic protein folding, protein protection, and storage. The bioprocessing environment is void of endotoxin, has low protease activity and secondary metabolite content, with a simple protein profile, aiding in downstream processing.” This produces pure, endotoxin-free growth factors, which Sloan says, leads to increased reproducibility in SC culture and aids in the transfer of protocols from preclinical to clinical trials.
Unlimited stem cells and possibilities
Induced pluripotent stem cells (iPSCs), which are derived from adult cells, instead of sourcing embryonic stem cells (ESCs), are one of the biggest advancements of this past decade according to Sloan. She says that iPSCs have the potential for personalized treatment, could be used for drug screening, and would reduce transplant rejection.
Stem Cell Media Search Tool
Search stem cell media
from different suppliers Search
With near limitless capabilities, companies are expanding their portfolios to include iPSC lines, reagents, and services. ATCC, for example, provides a number of these specialized products. “[We] offer over 20 human, footprint-free iPSC lines,” says Dezhong Yin, Ph.D., lead scientist at ATCC. This includes iPSCs derived from healthy donors and specific diseases such as Parkinson’s, cystic fibrosis, and Down syndrome. The full extent of their offerings can be found on their site, including a useful Stem Cell Culture Guide. “This guide provides the step-by-step instructions, tips, and techniques that laboratory workers need to routinely thaw, subculture, and cryopreserve iPSCs.”
Goldsborough notes that authentication is also important. She says that all ATCC cells have a historical STR profile and she recommends periodic mycoplasma and STR testing. “It is also vital to characterize the expanded cells at early and later passage to ensure they retain their pluripotency. A variety of methods are available including: flow cytometrical analysis of pluripotent marker expression, DNA microarray-based analysis of pluripotency, assays to test differentiation potential, and karyotyping.”
Challenges persist, but so do solutions
“Even with all the advances for stem cells, the culture of these cells is still demanding,” notes Yin. “Cells can easily differentiate in culture and require almost daily attention from skilled operators. Scale-up is particularly challenging as typical culture methods such as ‘cleaning’ the culture of any differentiated cells does not scale well especially to large-scale methods such as wave bags and bioreactors.”
Hilary Sherman, senior scientist at Corning Life Sciences, also cites limited expansion capabilities and issues with maintaining the quality of SCs throughout long-term passaging as examples of challenges in the field.
While 3D culture can have its own set of complications, Xie says that moving SCs to this system can be advantageous. “3D SC culture allows for complex spatial interactions between cells, extracellular matrix contact, and gradients of nutrition and oxygen, which better mimics the SC dynamic and specialized microenvironments in vivo.” An added benefit is that culturing SCs as spheroids does not require an attachment surface and is amenable to large-scale techniques such as stirred-tank bioreactors. For scientists looking into 3D options, which can allow for greater cell densities in a smaller footprint, the new Corning® Elplasia® plates are recommended.
Xie points out that spheroid culture of mesenchymal SCs results in “enhanced multidifferentiation potential, upregulated expression of pluripotency marker genes, and delayed replicative senescence.” These indicate enhanced stemness in the spheroids. Corning has protocols for working with its Spheroid Microplates and Matrigel. Of course, every cell line will require optimization.
Jacob says that there are critical details that scientists should be paying attention to when working with SCs including what to expect when looking through the microscope. “The morphology of iPSCs can vary among different cell lines. For example, the edges of the majority of iPSC colonies are smooth and round, but some iPSC colonies exhibit a spiky morphology. Some iPSC colonies differentiate at the center, others differentiate at the edges.” Other critical factors relate to confluence and handling time. Whatever direction you choose to take with your SC experiments, vendors can provide a wealth of information about specific lines and reagents. “[We] understand what’s needed for successful culture conditions.”